Initial goal: to miniaturize biomedical workflows and accelerate discovery |
Current state-of-the-art miniaturization
Water is piped from reservoirs to our homes through tubes with solid walls. How might one miniaturize reservoirs/pipes handling liquids in biomedicine? The usual way is to apply techniques used so successfully to shrink integrated circuits in computer chips [1].
|
|
A big drawback
But few biologists use the resulting 'chips' (right) – why? Reasons given include: they take days to make, they are expensive and complicated, their contents are inaccessible, and (probably the biggest reason) they are not made with cell-friendly materials [1]. |
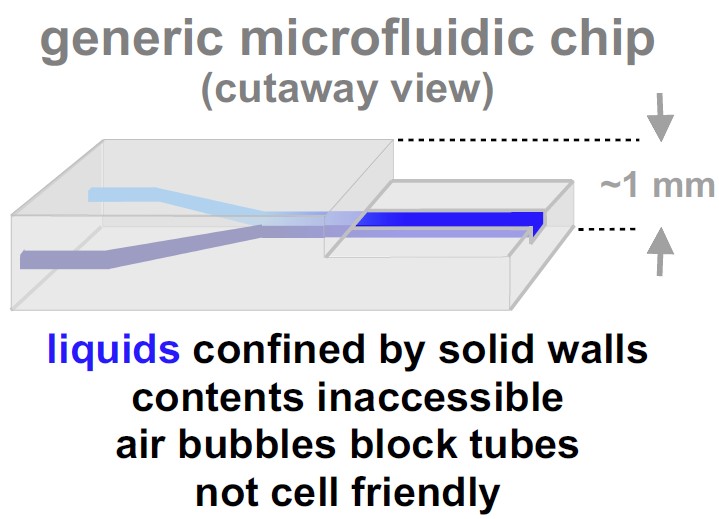 |
How do biologists grow cells? The answer is they use culture media and Petri dishes. Cell culture is difficult; that is why ~99% microbial species and most cell types in the human body have yet to be cultured successfully, and why biologists have spent decades finding the few cells and conditions currently used [2,3].
How might one build microfluidic devices using growth media and Petri dishes? The answer may sound silly, but we use fluid walls!
|
|
Five steps to a new and different solution
(1) Escape from the tyranny of gravity and reliance on solid walls
In the everyday world gravity is a powerful force, and water is always contained within solid walls – otherwise it just drains away.
In the micro-world, gravity becomes irrelevant – water striders skim over ponds, raindrops stick to windows.
Raindrops on the right defy gravity ‘pinned’ to the grass by powerful interfacial forces.
Air–water interfaces maintain drop shape. In other words, water is confined by fluid (not solid) walls. These walls are strong because they grip substrates so tightly. |
|
(2) Fluid walls can also confine complex microfluidic patterns
After drawing a complex pattern of growth medium plus blue dye on a 6 cm Petri dish, fluid walls easily maintain the pattern (right; letters have maximum widths / heights of ~500 / ~150 microns, respectively [4]). |
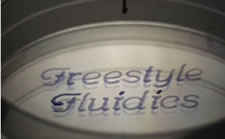 |
(3) Overlaying an immiscible liquid prevents evaporation
But small volumes soon evaporate (right, i); this is easily prevented by overlaying an immiscible oil (right, ii). We often use the fluorocarbon, FC40 (which is transparent, but shown green). This is arguably the most bio-inert [5] and non-sticky oil known (it is a close relative of Teflon). Now, a liquid wall of FC40 confines the drop.
|
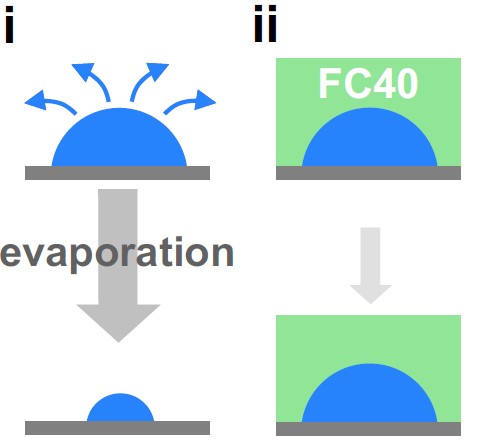 |
(4) FC40 walls similarly confine complex microfluidic patterns
The circuits below were ‘printed’ in air on 6 cm dishes, and overlaid with FC40. We are looking through the (transparent) oil.
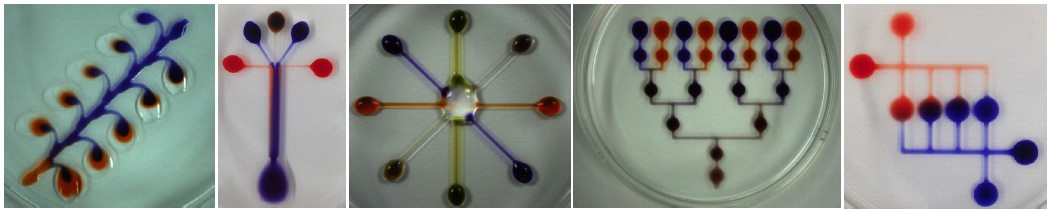
|
|
|
(5) Water can be added/removed without changing footprints
Consider a drop on a dish (middle on right). On adding water, drop footprint increases only when the advancing contact angle, θA, is exceeded. On removing water, it shrinks only when the receding contact angle, θR, is breached. Water is ‘pinned’ tightly to the dish!
For media under FC40, θA is >70° and θR is <3°; this big difference allows media to be added/removed without change in footprint.
In the example below, all chambers have identical footprints despite a ~30-fold variation in volume [6]. The range of working volumes is higher than that of wells in conventional microplates [6]!
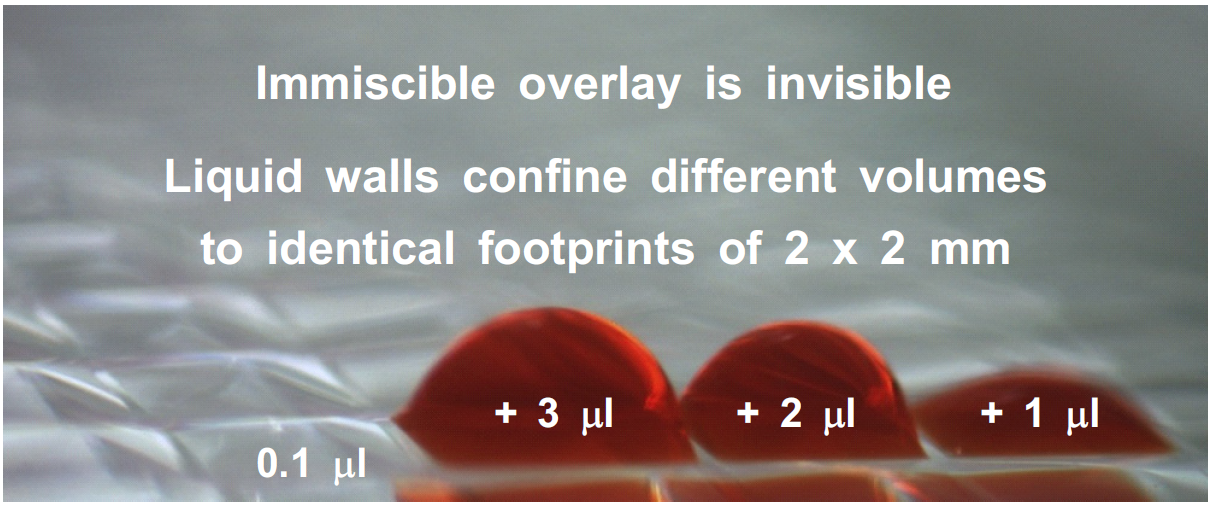
|
|
Steps 1-5 allow microfluidic arrangements to be built simply and quickly from Petri dishes and media. We have successfully used them to miniaturize many biomedical workflows including cell feeding, replating, cloning, cryopreservation, drug screening, lysis + RT-PCR, transfection + genome editing, and fixation + immunolabeling [4,6].
Fluid walls bring many benefits
• Built accurately in seconds – allows rapid prototyping (movie; movie).
• Robust – withstand violent agitation (movie).
• Morph above unchanging footprints – adapt to changing volumes.
• Biocompatible – made with media + FC40 on standard dishes.
• Transparent – see what goes on inside.
• Accessible – add/remove liquid through wall at any point.
• Simple interface between micro- and nano-liter scales.
• Fit seamlessly into current workflows – use existing microscopes,
incubators (FC40 is permeable to O2 + CO2), etc..
• Generate flow with external pumps – walls self-seal around
connecting tubes, and self-heal on withdrawal (movie).
• Generate flow without external pumps – exploit differences in
Laplace pressure (movie).
• Resistant to air bubbles causing catastrophic failures in conventional
'chips' – buoyancy expels bubbles through liquid walls (movie).
Fluid walls are built by:
• ‘Positive printing’ (draw circuit where wanted) – for flowing apps [4].
• ‘Reverse printing’ (draw walls where wanted) – for static apps [6,7].
|
|
Where we are in 2021
A review: Deroy, C., Nebuloni, F., Cook, P.R., and Walsh, E.J. (2021). Microfluidics on standard Petri dishes for bioscientists. Small Methods 2021, 2100724. [PubMed] [journal] [pdf]
|
|
Future goals
Our generic platform can be applied wherever small volumes are handled, and to other liquids/substrates. So watch this space…
Footnotes
1 Sackmann EK et al (2014) The present and future role of microfluidics in biomedical
research. Nature 507, 181-189.
2 Ling LL et al (2015) A new antibiotic kills pathogens without detectable resistance.
Nature 517, 455-459.
3 There seems to be little evidence for the oft-cited number of ~200 different human cell
types, and results from single-cell transcriptomics are rapidly increasing it.
4 Walsh EJ et al (2017) Microfluidics with fluid walls. Nat Commun 8, 816.
5 FC40 has been used as a blood substitute, and its close relatives for liquid ventilation of human preterm neonates.
6 Soitu C et al (2018) Microfluidic chambers using fluid walls for cell biology. Proc. Natl. Acad. Sci. USA 115, E5926-E5933.
7 Soitu C et al (2019). Raising fluid walls around living cells. Sci. Adv. 5, eaav8002. |
|
Top | Home | Maintained by Peter Cook |
|